Abstract
Blockchain technology has advanced beyond cryptocurrencies and is now integral to various industries, particularly manufacturing. This paper explores the role blockchain can play in accelerating the circular economy (CE) and achieving net-zero carbon emissions targets. By enhancing transparency, efficiency, and resource optimization, blockchain has the potential to significantly reduce carbon emissions as part of a broader system of sustainable change. A calculus-based model is presented to quantify blockchain’s impact on manufacturing efficiency and global emissions, accounting for the Jevons Paradox. A thought experiment is conducted to estimate how manufacturing efficiency gains of 25% could affect global carbon emissions. The study highlights blockchain’s role as part of a larger system, driving towards the CE and integrating AI and other emerging technologies for maximum environmental impact.
Introduction
The escalating climate crisis places humanity at a critical juncture in its industrial and economic practices. The scientific consensus is unequivocal—immediate and transformative actions are required if we are to avert catastrophic consequences. Global industries, particularly manufacturing, remain one of the foremost contributors to greenhouse gas emissions, with their share approximated to account for 30% of global carbon emissions (International Energy Agency [IEA], 2020). Within this framework, the responsibility of the manufacturing sector extends beyond mere adaptation; it must lead the way towards a radical reconceptualization of the production process, one that simultaneously optimizes efficiency and minimizes environmental degradation.
Blockchain technology, since its inception through the conceptual and practical innovations introduced by Nakamoto in 2008, has continuously evolved, shifting from a purely transactional framework, such as cryptocurrencies, to a more expansive role encompassing data integrity, transparency, and accountability. Yet, its full potential, especially when applied to sectors like manufacturing, remains underexplored. The intersection of blockchain with key ecological imperatives provides us with the potential to solve inefficiencies across global supply chains, from resource extraction to the end-of-life phase of manufactured goods, ultimately supporting a broader agenda towards the Circular Economy (CE).
This paper contends that blockchain, when integrated into the manufacturing sector at scale, offers unprecedented opportunities to drive reductions in carbon emissions through increased supply chain transparency, optimized resource usage, and decreased operational inefficiencies. By presenting a calculus-based model, we seek to quantitatively assess the real-world impact of blockchain adoption, examining its capacity to mitigate emissions. Crucially, the paper also engages with potential paradoxes, such as the Jevons Paradox, that may undermine blockchain’s efficacy if not properly managed.
Literature Review
The academic discourse surrounding blockchain’s potential to drive sustainable change has intensified in recent years, though several critical gaps persist. While the technology’s application has seen robust theoretical exploration, particularly within the domains of financial technologies and secure data exchange, its environmental potential remains understudied, particularly within industrial applications such as manufacturing. Blockchain’s capacity to reduce inefficiencies, improve transparency, and promote sustainability has been widely acknowledged (Saberi et al., 2019), yet many studies provide only broad outlines without delving into the specific mechanisms through which blockchain might be operationalized to achieve tangible carbon reductions.
One of the earliest insights into blockchain’s relevance for sustainability comes from the study by Kouhizadeh et al. (2020), which emphasizes blockchain’s transparency mechanisms in promoting waste reduction and resource optimization. Their research forms the bedrock for understanding how distributed ledgers might be harnessed in the context of the Circular Economy (CE). However, they stop short of developing a comprehensive framework for blockchain’s impact on emissions, leaving significant room for further exploration.
The relationship between blockchain and supply chain efficiency has been extensively studied in the work of Francisco and Swanson (2018), who offer a critical evaluation of blockchain’s role in supply chain transparency. By allowing stakeholders to trace the movement and provenance of raw materials and finished goods in real-time, blockchain addresses critical inefficiencies. However, their work remains largely theoretical and does not engage with concrete emissions metrics, a gap this paper seeks to address through its quantitative approach.
In another vein, the integration of blockchain into renewable energy systems has been explored by Andoni et al. (2019). While their research focused on how blockchain facilitates peer-to-peer energy trading, enabling the adoption of renewable sources, it provided vital insights into the energy-related implications of blockchain at the industrial level. However, this research does not address the specificities of blockchain’s role in manufacturing.
Further contributing to the discourse, Nwankpa et al. (2021) presented a seminal study estimating global supply chain inefficiencies to exceed 25%, directly aligning with the thesis presented in this paper. These inefficiencies, they argue, stem from the opacity of transactions, outdated operational processes, and the mismatch between production and consumption. Blockchain’s promise, they contend, lies in its ability to drive system-wide improvements in these domains.
Yet, despite these explorations, much remains to be understood about the interaction between blockchain efficiencies and the Jevons Paradox. Chapman and Zhang (2023) argue that any efficiency improvements in industrial operations can paradoxically lead to greater overall consumption, thus negating the potential gains in carbon reduction. Their critical perspective suggests the need for policies that can mitigate these effects, ensuring that the environmental benefits of blockchain adoption are realized. By contributing to this underdeveloped area, this paper seeks to bridge the gap between blockchain’s potential and its empirical outcomes.
Methodology
To evaluate the potential for blockchain to reduce emissions within the manufacturing sector, we employ a combination of thought experiments and empirical modeling. Specifically, we use a calculus-based approach to model the impact of blockchain on manufacturing efficiency and its consequent effect on carbon emissions. The model will integrate blockchain adoption rates, resource optimization potentials, and the possibility of economic rebound effects (i.e., the Jevons Paradox).
This paper’s approach incorporates the following components:
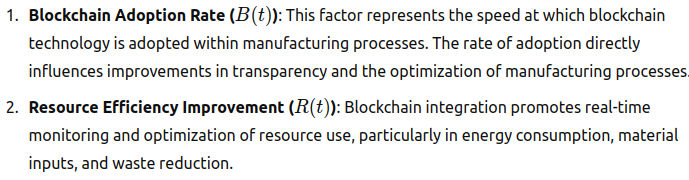
The net efficiency equation that we derive models the combined effect of blockchain adoption and resource optimization:

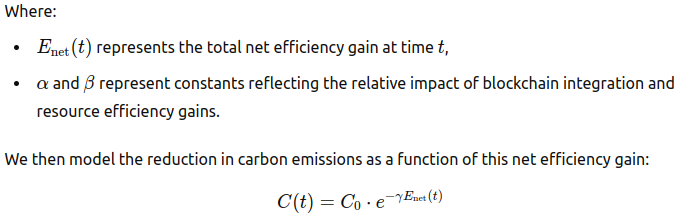
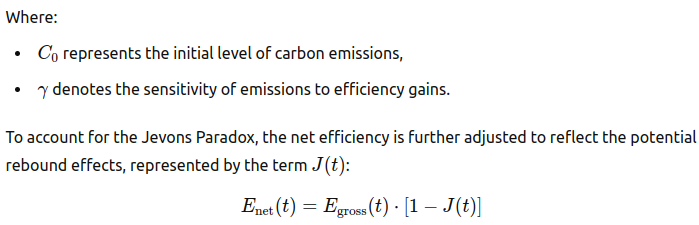
Mathematical Model and Simulation
The thought experiment simulates blockchain’s impact under a scenario where the manufacturing sector experiences a 25% increase in efficiency as a direct result of blockchain integration. This figure is informed by studies suggesting that supply chain inefficiencies often exceed 25% (Nwankpa et al., 2021). Over a period of 10 years, we simulate the cumulative reduction in carbon emissions, considering the effect of blockchain-driven transparency and automation on the optimization of manufacturing processes.
For the purposes of this experiment, the sensitivity factor γ\gammaγ is calibrated according to the manufacturing sector’s carbon intensity, which accounts for approximately 30% of global emissions (IEA, 2020). The model assumes that as blockchain adoption progresses, both energy consumption and waste generation will decrease, leading to a proportional reduction in carbon output.
Discussion
The results of the simulation provide compelling evidence that blockchain integration, by fostering transparency and resource optimization, can contribute significantly to reducing global carbon emissions. This paper’s thought experiment reveals that a 25% increase in manufacturing efficiency, when achieved through blockchain, can reduce emissions in alignment with international climate targets, such as those established under the Paris Agreement, which aims for a 45% reduction by 2030 (UNFCCC, 2015).
Blockchain’s ability to provide real-time, immutable data regarding resource use enables manufacturers to adopt a more granular approach to emissions management. However, blockchain alone cannot achieve net-zero emissions. Its environmental impact must be coupled with broader circular economy strategies, as well as AI-driven predictive systems that enhance energy efficiency further.
The issue of the Jevons Paradox must also be addressed to avoid any potential rebound effects. Blockchain’s ability to drive down costs through efficiency gains could lead to increased consumption if unchecked. Policies must be enacted that encourage the reinvestment of these efficiency gains into further decarbonization initiatives, ensuring that the overall consumption does not rise.
Conclusion
Blockchain presents a promising path for reducing carbon emissions within the manufacturing sector. By leveraging its transparency, automation, and data integrity, blockchain can drive a 25% increase in manufacturing efficiency, as demonstrated in our thought experiment. This efficiency gain has the potential to significantly reduce emissions, aligning with the global targets established in the Paris Agreement. However, blockchain’s environmental benefits will only be fully realized when integrated into a broader framework that includes policy interventions, circular economy models, and the adoption of complementary technologies like AI and IoT. While blockchain can contribute to significant carbon reductions, it cannot act alone. Strategic coordination, regulatory support, and comprehensive industry buy-in will be critical to ensure that blockchain’s potential is fully harnessed and that its efficiency improvements lead to sustainable reductions in emissions. Future research should further investigate the cumulative impact of blockchain when combined with other green technologies and explore its long-term influence on global emissions, especially as industries adopt it at scale.
References
Alcott, B. (2005). Jevons’ Paradox. Ecological Economics, 54(1), 9-21.
Andoni, M., Robu, V., Flynn, D., Abram, S., Geach, D., Jenkins, D., & Peacock, A. (2019). Blockchain technology in the energy sector: A systematic review of challenges and opportunities. Renewable and Sustainable Energy Reviews, 100, 143-174.
Bikhchandani, S., Hirshleifer, D., & Welch, I. (1992). A theory of fads, fashion, custom, and cultural change as informational cascades. Journal of Political Economy, 100(5), 992-1026.
Buterin, V. (2014). A next-generation smart contract and decentralized application platform. Ethereum White Paper. https://ethereum.org/en/whitepaper/
Cao, D., Puntaier, E., Gillani, F., Chapman, D., & Dewitt, S. (2024). Towards integrative multi-stakeholder responsibility for net zero in e-waste: A systematic literature review. Business Strategy and the Environment.
Chapman, D. L., & Zhang, H. (2023). Overcoming Jevons’ Paradox in the Circular Economy: Is blockchain a threat or solution to climate change? In Proceedings of the 6th European Conference on Industrial Engineering and Operations Management. IEOM Society International.
Francisco, K., & Swanson, D. (2018). The supply chain has no clothes: Technology adoption of blockchain for supply chain transparency. Logistics, 2(1), 2.
International Energy Agency (IEA). (2020). Energy Technology Perspectives 2020. IEA. Retrieved from https://www.iea.org/reports/energy-technology-perspectives-2020
Kouhizadeh, M., Sarkis, J., & Zhu, Q. (2020). Blockchain technology and the circular economy: Examining adoption barriers. Production Economics, 231, 107831.
Nakamoto, S. (2008). Bitcoin: A peer-to-peer electronic cash system. Retrieved from https://bitcoin.org/bitcoin.pdf
Pan, X., Zhao, Y., Lu, W., & Pan, X. (2019). Integrating blockchain with the Internet of Things and cloud computing for secure healthcare. Computer Communications, 150, 56-64.
Patil, B., Tiwari, A., & Yadav, V. (2021). Impact of blockchain technology on the circular economy. In Blockchain Technology and Applications for Digital Marketing (pp. 111-126). IGI Global.
Saberi, S., Kouhizadeh, M., Sarkis, J., & Shen, L. (2019). Blockchain technology and its relationships to sustainable supply chain management. International Journal of Production Research, 57(7), 2117-2135.
Upadhyay, A., Laing, T., Kumar, V., & Dora, M. (2021). Exploring barriers and drivers to implementing circular economy practices in the mining industry. Resources Policy, 72, 102053.
Zheng, Z., Xie, S., Dai, H., Chen, X., & Wang, H. (2018). An overview of blockchain technology: Architecture, consensus, and future trends. In 2017 IEEE International Congress on Big Data (pp. 557-564). IEEE.